MPD: Ionized Gas & Plasma Thrust
- Sylvia Rose
- Mar 21
- 4 min read
Magnetoplasmadynamic (MPD) thrusters use ionized propellants like xenon, argon, and krypton to generate thrust through plasma acceleration.
They far surpass current limits of fuel consumption and attainable velocities.
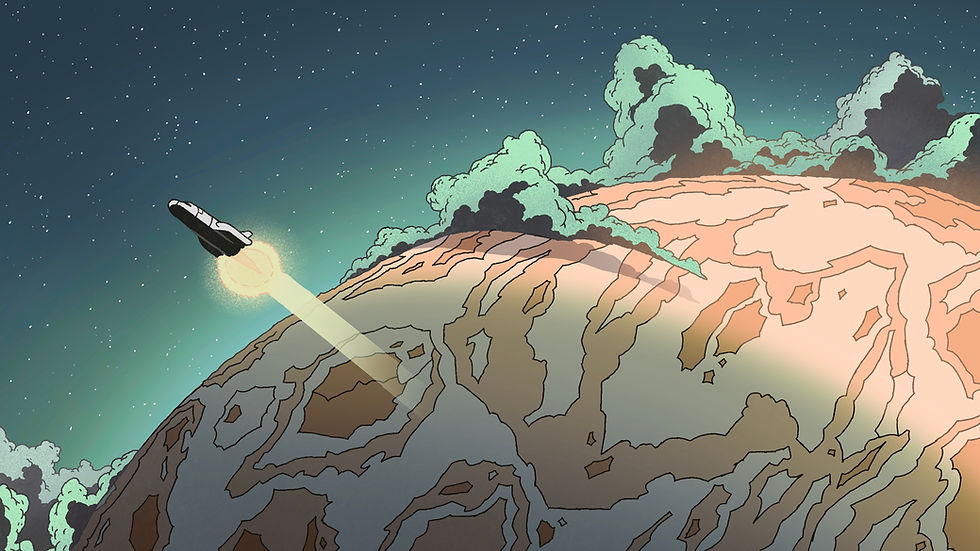
MPD thrusters manipulate ionized gas, or plasma, using electromagnetic fields. Examples of ionized gas include lightning, fluorescent lighting and the aurora borealis or northern lights. Chemical rockets operate at around 450 seconds specific impulse. An MPD thruster achieves over 5000 s.
Ionized gas occurs throughout the universe. It's especially abundant near hot stars, within galaxies, and in the diffuse interstellar medium, as well as in the Earth's ionosphere.
The diffuse interstellar medium (ISM) encompasses low-density, gaseous and dusty regions between stars within a galaxy. It contains primordial leftovers, stellar detritus, and materials for future stars and planets.
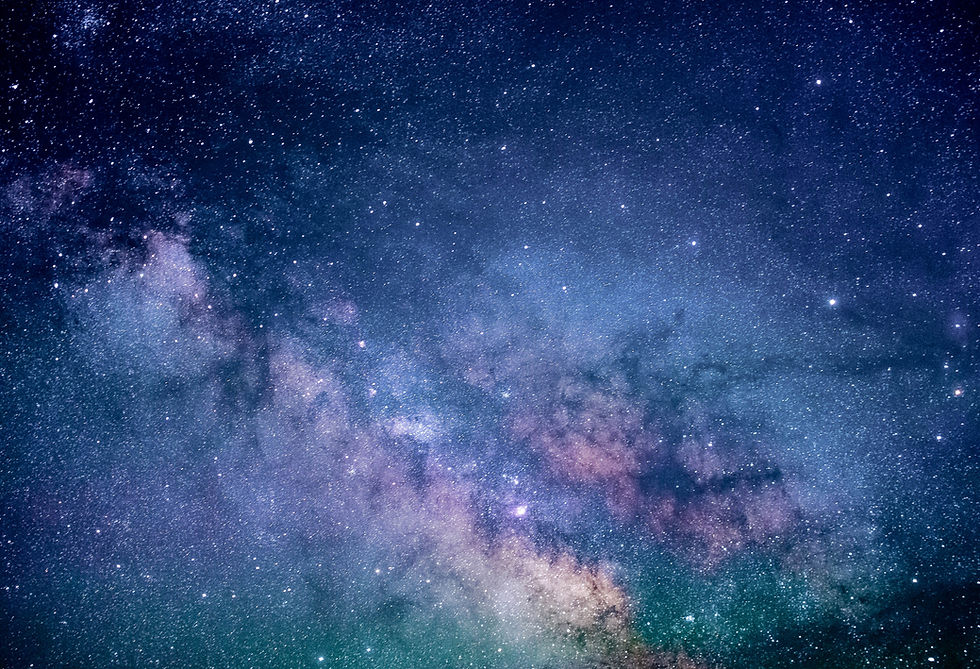
Ionization: Creating the Supercharged State
The first step is to create a plasma. Electrons are removed from the atoms of a propellant gas, typically a noble gas like xenon, argon, or krypton. The gases are have low ionization energy and high atomic mass.
Loss of electrons from an atom needs energy input, such as heat. The energy required to remove an electron from a neutral atom is the ionization energy of that atom.
When heating a gas to extreme temperatures the atoms become agitated and collide with incredible force. Eventually, the energy is enough to knock electrons loose, leaving positively charged ions and free-floating electrons.

This mixture of ions, electrons, and neutral atoms (if ionization isn't complete) constitutes the plasma.
Methods used for ionization include
Radio Frequency (RF) discharge: Applying a radio frequency electromagnetic field to the gas.
Direct Current (DC) discharge: Passing a high-current electrical discharge through the gas.
Microwave energy: Microwave plasma or discharge is generated by applying microwave energy, electromagnetic waves with frequencies between 300 MHz and 300 GHz), to a gas.

Electromagnetic Acceleration
Once the plasma is created, the MPD thruster begins acceleration through electromagnetic forces. The combination of electric and magnetic fields creates power.
Electric Field
High voltage is applied between an anode (positive electrode) and a cathode (negative electrode) in the thruster. The ensuant electric field exerts force on the charged particles in the plasma. The positively charged ions are pushed away from the anode, toward the cathode.
Magnetic Field
A strong magnetic field is generated within the thruster using a powerful electromagnet coiled around the thruster axis. This magnetic field interacts with the electric current flowing through the plasma, creating a Lorentz force.
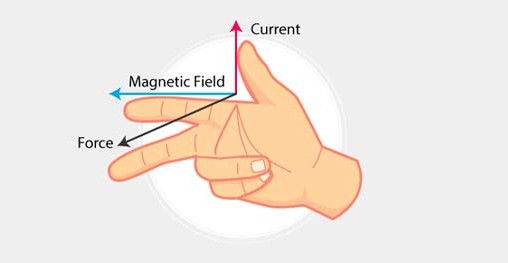
The Lorentz force acts perpendicular to both the electric current and the magnetic field. It pushing the plasma in a specific direction, out the back of the thruster.
The electric field is the 'gas pedal,' accelerating the ions, while the magnetic field is like a steering wheel directing their momentum. The combination of these forces optimizes acceleration of the plasma.
Dual Field Approach
Most MPD thruster designs feature both electric and magnetic fields inside the thruster chamber. The electric field is generated through applied voltage across electrodes. The magnetic field comes from electric current flowing through coils surrounding the chamber.

Generating Thrust
As ions gain acceleration in their plasma state, they exit the thruster at high speed. According to Newton's Third Law, every action prompts an equal and opposite reaction.
Thus, as ions are expelled, a thrust force is produced in the opposite direction, pushing the spacecraft forward. MPD thrusters have several advantages
Higher Exhaust Velocity: Plasma can be accelerated to much higher velocities than combustion products in chemical rockets, leading to improved fuel efficiency and potential for greater ultimate velocities.
Variable Thrust: The thrust of an MPD thruster can be easily adjusted by varying the voltage and current applied, for precise maneuvering and trajectory control.

Higher Specific Impulse: Specific impulse is a measure of how efficiently a rocket engine uses propellant, and MPD thrusters can achieve significantly higher specific impulses compared to chemical rockets. This translates to longer mission durations and greater payload capacity.
Reduced Toxicity: The use of inert gases like xenon or krypton minimizes risk of environmental toxicity, a safer alternative to usual rocket fuels. For instance, use of liquid hydrogen carries risks of combustion or toxicity. The inert nature of krypton enables more stable operation.
Extended Operational Life: MPD thrusters have less wear and tear than do traditional engines. This translates to longer operational life and lower maintenance costs, a financial bonus for extended missions.
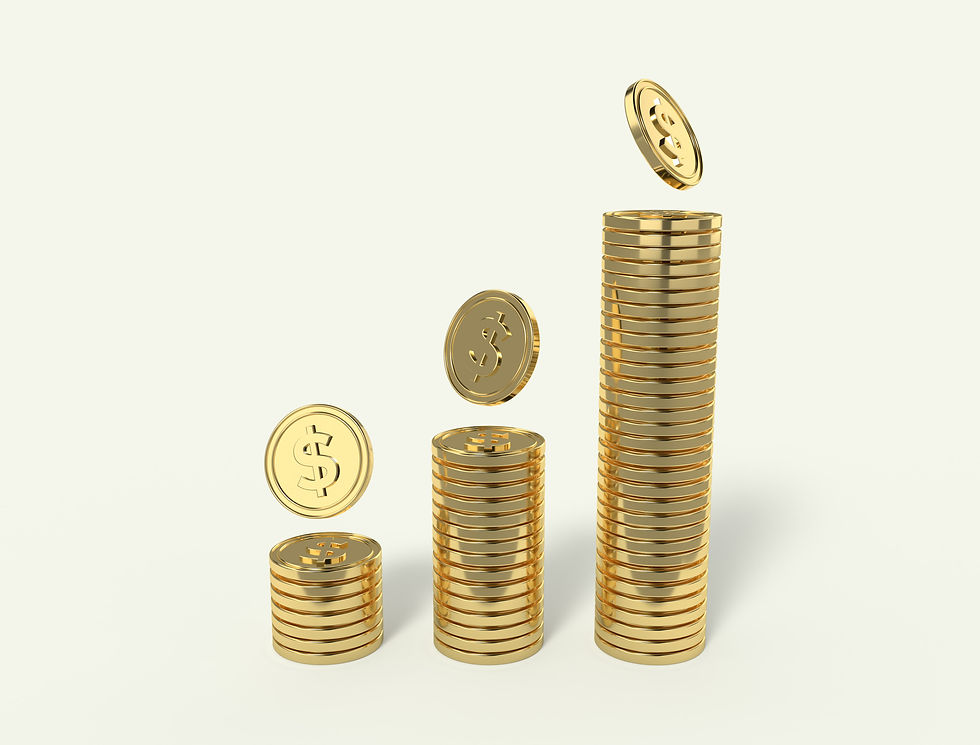
Problems
Despite their potential, MPD thrusters have a few drawbacks. They need substantial power input, which means large and heavy power sources. Overheating and electrode erosion are also concerns.
The intricate systems needed to create and manage electromagnetic fields complicate design and deployment of MPD thrusters. Engineers must consider many factors to ensure reliable operation in diverse conditions.
Real Time Space Use
Experimental prototypes are first flown on Soviet spacecraft. An MPD thruster is tested on board the Japanese Space Flyer Unit as part of EPEX (Electric Propulsion Experiment).
Launched March 18, 1995, it's retrieved by space shuttle mission STS-72 January 20, 1996. To date, it is the only operational MPD thruster to fly in space as a propulsion system. R&D continues.
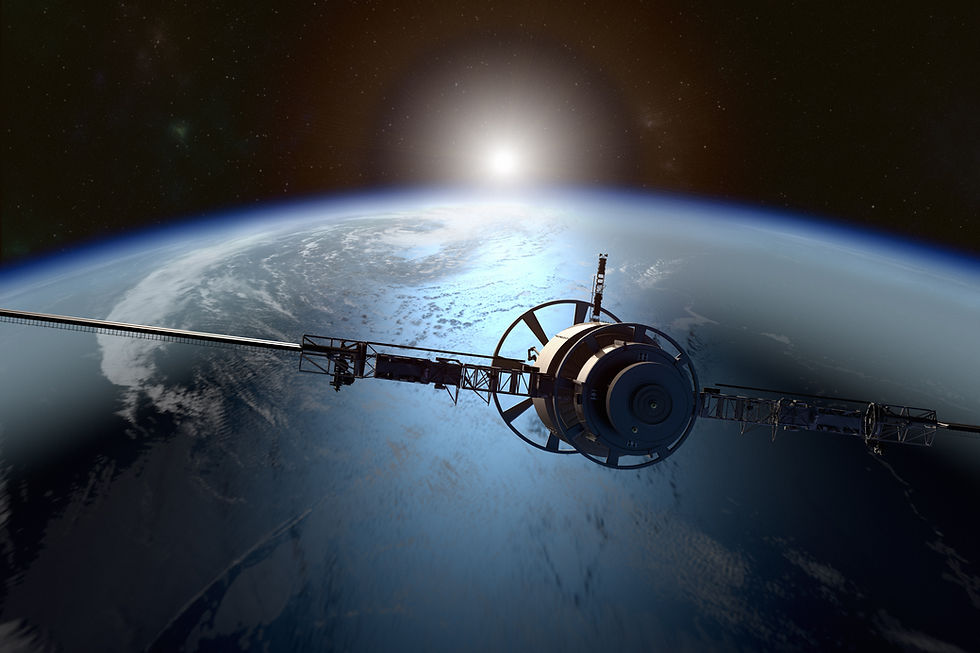
READ: Lora Ley Adventures - Germanic Mythology Fiction Series
READ: Reiker For Hire - Victorian Detective Murder Mysteries